Temperature is a key factor in plant growth and development. Along with the levels of light, carbon dioxide, air humidity, water and nutrients, temperature influences plant growth and ultimately crop yields. All these factors should be in balance. Temperature influences the plant in the short term as well as the long term.
Not surprisingly, a great deal of research work has been done into proper temperature strategies for efficient greenhouse production. However, the optimum temperature for a plant depends on a range of factors. A plant’s reaction to the atmospheric temperature around it depends on which stage of development that plant is in. Plants have a kind of biological clock which determines their sensitivity to temperature.
Differences between air temperature and plant temperature
Most biological processes will speed up at higher temperatures, and this can have both positive and negative effects. For example, faster growth or fruit production is one benefit, in most cases. However, the excessive respiration that occurs is adverse because it means that there is less energy for fruit development and the fruits will be smaller. Some effects are short term, while others are longer term. The plant’s assimilation balance, for example, is influenced by the temperature and is affected immediately. Flower induction, on the other hand, is determined by the climate over a much longer period.
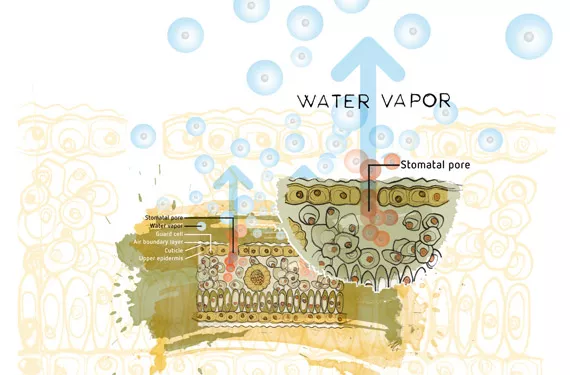
Plant temperature and air temperature are not equal because plants are able to cool off through evaporation and warm up through irradiance. Plants seek to reach their optimal temperature, and a balance between air temperature, relative humidity and light is important in this. If light levels are high, the plant will heat up, resulting in a difference between plant temperature and air temperature. To cool down, the plant’s transpiration rate must increase. As well as temperature, the transpiration rate depends on environmental conditions such as light, the level of atmospheric CO2 and relative humidity, but also on the plant species.
Vapour Pressure Deficit
The relative humidity of the environment depends on temperature and wind speed. Higher temperatures generally lead to increased transpiration. When there is no air movement, the air around the leaves will become saturated with water vapour, slowing down the process of evaporation. If the air is saturated with water, a film of water will condense on and around the leaves providing a good environment for pathogens, which can attack the plant.
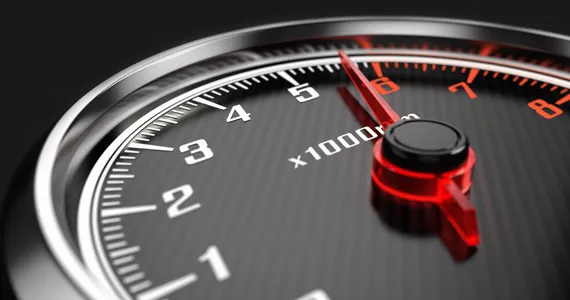
The difference in water vapour content between the air and the saturation point is called the vapour pressure deficit (VPD). The higher the VPD, the more water the plant can give off through transpiration. However, if the VPD is too great, the plant can become stressed because it is unable to replace the amount of water which it is losing through transpiration. This does not cause a problem for short periods – the plant will absorb enough water the following night to recover. But when the VPD stays high for a longer period, the plant is unable to recover the next night and irreversible plant damage such as burned leaves or petals can occur.
Leaf thickness measurements give a visual impression of the potential of a plant to recover. The leaves actually become thinner during the day because they lose water through transpiration, but when a leaf is thinner on one night than it was the previous night, this is a sign that the plant has failed to recover.
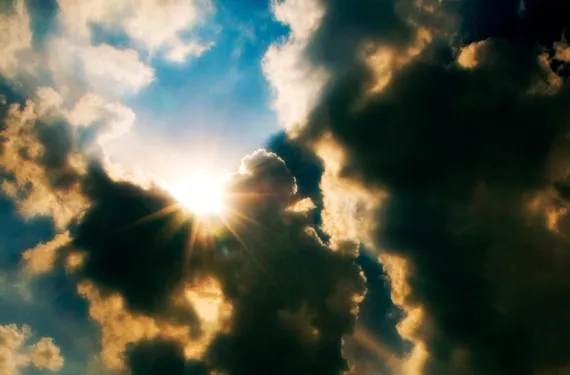
Stomata
Plants are able to regulate the process of transpiration and cooling by using specialised plant organs called stomata. The stomata are specialised cells in the leaves which can open or close, limiting the amount of water vapour that can evaporate. The higher the temperature rises, the more the stomata will evaporate when they are open.
Also other environmental factors influence the rate at which this process (stomatal conductance) occurs – for example, higher relative humidity leads to more rapid conductance, while higher CO2 levels will depress the rate of stomatal conductance. But conductance is also influenced by factors other than environmental ones, such as plant hormones and the colour of the light (the wavelength)that the plant is receiving.
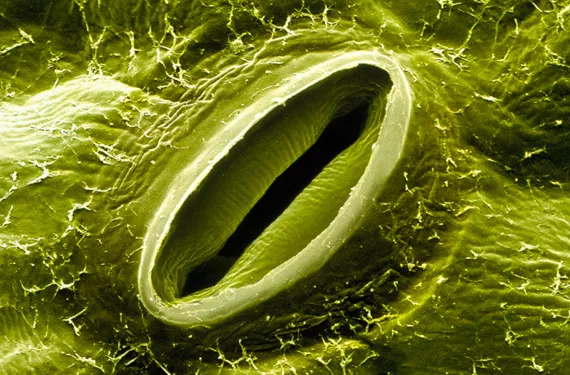
Optimum day and night temperatures
Different processes occur in the plant during the day and at night, and the optimum temperature for the plant will differ accordingly. The transportation of sugars occurs mostly during the night and mainly towards the warmer parts of the plant. The leaves cool faster than the fruits and flowers, and therefore most of the available energy goes to these parts of the plant, which need the energy to grow and develop. The ability of the plants to ‘distinguish’ between temperature variations during the day and night is called thermoperiodism, and it has an effect on flowering, fruiting and growth.
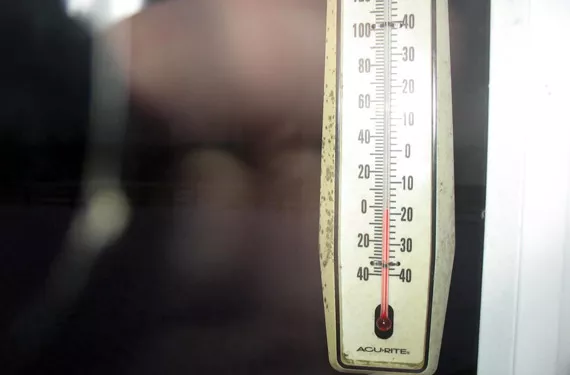
The optimum air temperature also depends on the light intensity and the amount of carbon dioxide in the air. Plants function in a similar way to cold-blooded animals, in that their metabolism and the rate of photosynthesis increase in line with the ambient air temperature. When temperatures are very low (how low depends on the plant variety), hardly any photosynthesis will occur, regardless of how much light there is. The rate of photosynthesis increases as the air temperature rises. When light and temperature are in balance, the level of ambient CO2 will be the limiting factor. If there is enough CO2 available, the rate of photosynthesis will increase as the temperature rises, although other factors do also play a role, such as the enzyme RuBisCo.
Drop and temperature integration (DIF)
The concept of DIF concerns the relationship between day and night-time temperatures. The effects of diurnal temperature alternation on the lengthways growth of plant stems depends on the difference (DIF) between day and night-time temperatures (which is calculated by subtracting the night-time temperature from the day-time temperature), rather than on separate and independent responses to day and night-time temperatures. In other words, it is this temperature difference that is important, as well as which is higher – the night temperature or the day temperature.
The growth of foliage is not greatly affected by DIF, but the growth of the internode stem sections is affected. Plants grown under a positive DIF are taller than plants grown at a zero DIF, and plants grown under a zero DIF are taller and have longer internode sections than plants grown under a negative DIF. Other important morphogenetic responses to negative DIF (i.e. when the day-time temperature is lower than the night-time temperature) include shorter petioles, flower stems, flower peduncles and leaves.
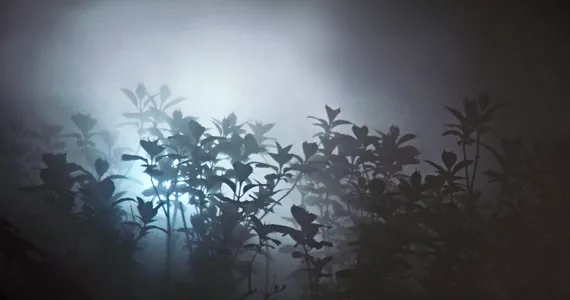
The variation in the sensitivity of stem elongation to temperature within the day period and night period may be controlled by an endogenous growth rhythm. A circadian growth rhythm (lasting about 24 hours) was identified in 1994 in Chrysanthemum. Plant stem elongation is not constant during a 24-hour light and dark cycle. Both short-day plants and long-day plants grown under flower-inductive conditions elongate more rapidly during the night than the day. Orchids need a period of low night temperature to flower.
Air temperature is a primary environmental factor that affects plant development and growth rate. However, air temperature is never an isolated issue. Every factor in plant growth interrelates with every other factor and the challenge is to find any weak link in the chain. This article has explored many of these factors, but there are still others which are just as important, such as water balance and therefore, indirectly, transpiration. Everything that is or will occur in the plant does so under the first control point of air temperature; getting this right is the first step on the long road to successful crop production.